Oncomedicine 2018; 3:110-116. doi:10.7150/oncm.22098 This volume Cite
Review
The role of E2F transcription factors family in Urothelial Carcinoma
1. First University Urology Clinic, Laiko Hospital, University of Athens, 17, Ag.Thoma Str, GR11527, Athens, Greece
2. Molecular Carcinogenesis Group, Department of Histology and Embryology, School of Medicine, National Kapodistrian University of Athens, 75 Mikras Asias Str, GR11527, Athens, Greece
3. Bone Marrow Transplant Unit, Haematology-Lymphoma Clinic, Evangelismos General Hospital, 45-47 Ypsilantou Str, GR10676, Athens, Greece
Abstract
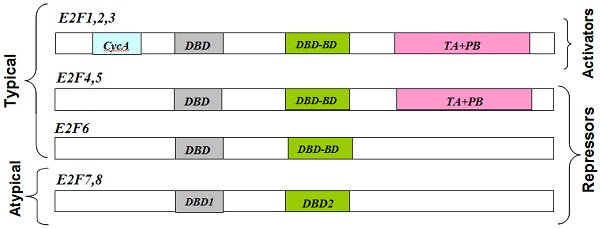
E2F transcription factor family is a group of 8 proteins that interact with pocket proteins and bind to target DNA in order to modulate cell cycle progression. Thus, their role is pivotal to cancer development and progression. Bladder cancer, a prominent cancer that affects both sexes, is also affected by E2F. E2F-1, E2F-3 and E2F-4 and -5 have a distinct impact on bladder development, progress and prognosis. However the role of the remaining E2Fs remains obscure, awaiting newer data that could provide useful therapeutic targets.
Keywords: E2F transcription factor, cancer, bladder, apoptosis, regulation
Introduction
Urothelial carcinoma, also known as transitional cell carcinoma (TCC) or just plain bladder cancer is by far the most common type of bladder cancer, accounting for almost 90% of all cases of bladder cancer. It arises from the urothelium of the bladder, but since urothelial cells line other parts of the urinary tract, it is not uncommon that patients with bladder cancer sometimes also present tumours to the renal pelvis, the ureters and the urethra [1]. It affects both men and women (3:1 ratio) being most prevalent to Caucasians than to Asians or Blacks. In 2012, bladder cancer was the ninth most frequent cancer worldwide (in both sexes) and the 13th most common cause of cancer death [2].
Bladder cancer is a chronic disease, affected by both genetic and environmental parameters, and some of the risk factors associated with the development of the initial tumour may influence its progress and final outcome. Smoking and chronic inflammation are the most studied environmental factors. Chronic infection with Schistosoma haematobium, following exposure to infested water, particularly in Africa and the Middle East, is a probable cause, while exposure to industrial chemicals such as metalworking fluids is also implicated in bladder cancer [3].
E2F is a group of genes, spread throughout the genome, encoding for basic helix-loop-helix transcription factors that control expression of a variety of genes involved in cell cycle progression.
There are several studies on E2F on bladder cancer development and prognosis, however there are no definite conclusions on their individual impact nor any mechanism of their action. This is due partially to the complexity of cancer development and partially to the wide range of action of E2F family. In this short review, we will present all the current knowledge on E2F involvement in bladder cancer development and progression and we will discuss potential therapeutic targets based on these findings.
E2F family of transcription factors
To date, eight E2F genes, producing 10 distinct E2F proteins, have been identified that exhibit varying degrees of sequence and structural differences, but share a very well conserved DNA binding domain [4]. E2F transcription factors typically form heterodimeric complexes with transcription factors (through a partner protein domain) and also have N-terminal DNA binding and dimerization domains (Fig.1). E2F transcription factors can act as mediators of transcriptional activation (E2F 1-3) or repression (E2F 4-8) (Table 1). Among E2F transcriptional targets are cyclins, cyclin dependent kinases (CDKs), checkpoints regulators, DNA repair and replication proteins. DNA microarray analysis reveals unique sets of target promoters among E2F family members suggesting that each protein has a unique role in the cell cycle, but there is a great deal of redundancy among them [5].
Normally, the Rb pathway constitutes a central molecular module involved in the regulation of cell cycle progression, while in cancer cells, alterations in this pathway promote a faster cell cycle and drive uncontrolled tumour growth [6, 7]. In non-proliferating cells, Rb protein remains hypophosphorylated in the nucleus and forms Rb-E2F repressor complexes to inhibit the expression of genes that promote S phase entry; additionally, it directly binds to and inhibits the activity of E2F activators (E2F-1, E2F-2, E2F-3). Specifically, during the G0 phase, expressed E2F-4 and E2F-5 limit the expression of E2F-targets which promote entry into the G1 phase of the cell cycle. Mitogenic signalling results into the phosphorylation of Rb protein by cyclin-dependent kinases. This renders Rb protein inactive, directs the release of the E2F repressors and results into the increased expression of the activators E2F -1, -2 and -3 at the end of this phase. The presence and action of these activators on cognate target genes, triggers a transcriptional programme driving cells into S phase; the action of the repressors E2F-6, E2F-7 and E2F-8 brakes the S phase, while E2F-7 and E2F-8 can also specifically mediate the repression of E2F activators. Without this braking mechanism E2F-11 proteins would continue to the activation of genes encoding transcriptional components and aberrant DNA replication [4].
Schematic representation of E2F transcription factors (N-to-C direction). Note that the typical E2Fs have DP-binding domains, which are replaced by a second DBD in the atypical E2Fs [Adapted from [54]]. CycA: cyclin A-binding domain; DBD: DNA-binding domain; DP-BD: Dimerization-binding domain; TA+PB: transactivation and pocket protein-binding domains.
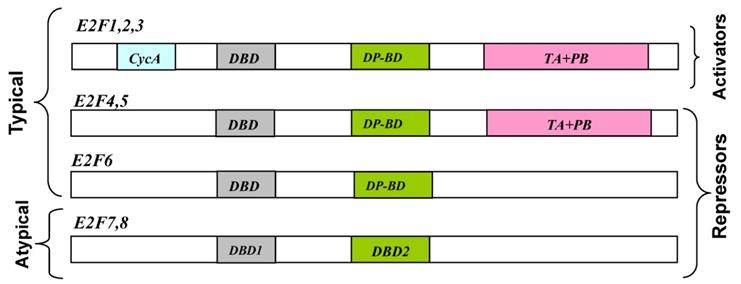
Summary of E2F localization and action in urothelial carcinoma [52].
Factor | Localization | Cell cycle | General action | Bladder cancer | Reference |
---|---|---|---|---|---|
E2F-11 | Nucleus | G1/S-phase | Induction | Overexpression | Hayami et al., 2010 |
Decreased expression | |||||
E2F-21 | Nucleus | G1/S-phase | Induction | Overexpression | Hayami et al., 2010 |
E2F-3a2 | Nucleus | G1/S-phase | Induction | Overexpression | Hurst et al., 2008 |
E2F-3b2 | Nucleus | G0/G1 phase | Suppression | Hurst et al., 2008 | |
E2F-43 | Cytoplasm | G0/G1 phase | Suppression | Translocate to nucleus | Parisi et al., 2009 |
E2F-54 | Cytoplasm/ | G0/G1 phase | Suppression | Translocate to nucleus | Umemura et al., 2009 |
E2F-65 | Nucleus | G1/S phase | Suppression | Yang et al., 2007 | |
E2F-76 | Nucleus | S-phase | Suppression | Weijts et al., 2012 | |
E2F-86 | Nucleus | G1/early S-phase | Suppression | Weijts et al., 2012 |
The inactivation of the retinoblastoma (Rb) pathway is a common occurrence in most human cancers. The main view for carcinogenesis in most human cancers, is that inactivation of Rb results in disassembly of Rb-E2F co-repressor complexes, alleviating the repression of genes that are necessary for progression through the cell cycle. Furthermore, the dissociation of hyperphosphorylated Rb from E2F activators leads to the inappropriate accumulation of free E2F-1, E2F-2 and E2F-3 with unmasked transactivation domains, resulting in additional transactivation of these genes. According to this prevailing model, transcriptional regulation by canonical E2Fs is controlled through association with the pocket proteins, i.e. the retinoblastoma (Rb) family of tumour suppressor proteins (pRb, p107 and p130), in the case of E2F 1-5, or in the case of E2F-6, with the epigenetic silencing polycomb group (PcG) [8]. p130 and p107 mainly interact with E2F-4 and E2F-5, while pRb preferentially binds E2F 1-3 [9]. These associations facilitate recruitment of histone deacetylases and methyltransferases to target promoters and subsequent transcriptional repression. Phosphorylation of Rb by CDK 4/6 results in the disruption of the Rb-E2F repressor complexes, unleashes E2F activity, thus modifying target gene transcription and entry of cells into the S phase [10].
In bladder tumours with complete loss of Rb function, it has been known for some time that the locus of E2F3 is amplified [11]. Similar data have been reproduced in Rb-knockout mice which showed low expression of p53 protein in bladder tumour tissue, with E2F-3 being highly expressed in tumour-tissue of wild-type mice and significantly upregulated in Rb-knockout mice, hinting at the connection between Rb deficiency and E2F-3 expression enhancement, leading to urinary bladder cancer progression [12]. Gene expression profiling of resected bladder tumours, revealed that E2F-1 expression was up-modulated in invasive tumours while expression was low in superficial tumours, suggesting a possible role of E2F-1 as an indicator of progression from the superficial to the invasive phenotype [13]. Data showed that E2F-1 exerts its role through its downstream effector EZH2 that is found overexpressed, along with E2F-1, in invasive bladder cancer [13]. Surprisingly, a study on the potential of CDK4/6 as a therapeutic target within the Rb pathway, where treatment with cyclin CDK4/6 inhibitors PD-0332991 or LY2835219 was tested on ten bladder cancer cell lines, resulted in decreased E2F target gene expression and cell cycle progression from G0/G1 to the S-phase, without affecting apoptosis [14].
E2F-1 is the prominent and most studied of all E2F factors. E2F-1 drives cell cycle progression at the G1- to S-phase boundary; however, overexpression of E2F-1 can induce apoptosis. E2F-1 is a remarkable example of a network hub as it interacts with many genes, proteins, and other transcription factors, through a variety of regulatory mechanisms. In the context of solid tumours, unbalanced E2F-1 regulation can lead to the emergence of aggressive tumour cells, thus driving fast cancer progression, resistance to therapy and metastasis formation.
It is now known that E2F-1 drives epithelial-mesenchymal transition (EMT), similar to the classical EMT inducer TGFB1, via signaling pathways that involve non-coding RNAs [15]. EMT is a biologic process that allows a polarized epithelial cell, to 'transform' into a mesenchymal cell phenotype, with enhanced migratory capacity, invasiveness, elevated resistance to apoptosis. As the excessive epithelial cell proliferation and angiogenesis are hallmarks of the initiation and early growth of primary epithelial cancers, the subsequent acquisition of invasiveness, is believed to herald the onset of the last stages for metastatic dissemination, with life-threatening consequences.
Indeed, enforced E2F-1 expression in advanced tumours and metastases of different kinds of cancers correlates with pronounced resistance towards therapy and poor patient prognosis [16] and clinical results indicate that E2F-1 is upregulated in high-grade bladder [16].
Khan et al. (2017) examined the effects of E2F-1 activity on the invasive capacity of patient-derived metastatic bladder tumour cell lines using functional invasion assays, western blotting, and PCR analysis and revealed a clear correlation of E2F-1 expression with the invasive behavior and EMT marker expression in invasive bladder (UM-UC-3, SW1710, J82, T24) vs. low expression in non-invasive or less-invasive epithelial bladder (RT-4, VM-CUB1, HT1197) cell lines [17].
In bladder cancer cell cultures, E2F-1 protein levels increased following treatment with the DNA damaging agents suggesting that E2F-1 may act in p53-independent fashion [18]. Higher expression of both E2F-1 and E2F-2 in bladder tumour tissues than in non-neoplastic tissues has been demonstrated both in vitro and in vivo, possibly through the mechanism of histone modification of the chromatin [19]. Aristolochic acid, a plant component commonly used in Chinese herbal medicine, has been implicated in urothelial carcinoma, since it increases Rb/E2F-1 complex which prevents the release of E2F transcription factor, leading to accumulation of cells in the G0/G1 phase [20]. However, immunohistochemical detection of nuclear E2F-1 protein in cystectomy cancer samples revealed that E2F-1 was not a significant independent predictor of pelvic recurrence, metastasis nor death [21]. Moreover, in urothelial bladder cancer cell lines, the effectiveness of the chemotherapeutic antimetabolite drug 5-fluorouracil (5-FU) depended, among other factors, on the Rb phosphorylation and E2F-1 expression [22].
E2F-3 encodes two proteins, E2F-3a and E2F-3b, through the use of alternative promoters and different 5′-coding exons. These share DNA binding, heterodimerisation and pocket protein binding domains and differ only in their N-termini. Their function is not entirely clear, but data suggests that they may have partially opposing roles: the E2F-3a is classified as an activator, expressed in proliferating cells with peak levels in late G1; while E2F-3b has been classified as a repressor E2F, and is constitutively expressed throughout the cell cycle [11]. E2F-3a is the predominant family member involved in subsequent G1-to-S phase transitions and has a unique role in centrosome duplication, while E2F-3b has been shown to preferentially bind pRb and repress S-phase genes [23]. Deregulated E2F-3 appears to be an important driver of proliferation in bladder carcinomas, since amplification of E2F-3 is particularly common in this type of cancer. Interestingly, genomic studies have shown that tumours that amplify E2F-3 often also acquire mutations in RB1, suggesting that there may be continuous selection in these tumours for changes that sequentially elevate E2F-3 activity [24]. Amplification and overexpression of the E2F-3 gene at 6p22 in human bladder cancer is associated with increased tumour stage, grade and proliferation index [25].
E2F-4 is ubiquitously expressed throughout cell cycle, accounts for most of the E2F endogenous activity and is primarily localized to the cytoplasm in its free form [26]. E2F-4 and E2F-5 mRNA expression is maximal in mid-G1 phase before E2F-1 expression is detectable, suggesting a contribution of these proteins in the regulation of early G1 events including the G0/G1 transition. When bound to the pocket proteins, in G0/G1 phase, E2F-4 accumulates in the nucleus where it actively represses E2F-target genes [27]. Loss of function of both Rb and E2F-4 has been observed in the urothelium transitional epithelium of both low- and high-grade carcinomas [28]. E2F-4 expression level is a useful predictive marker for the effectiveness of intravesical BCG therapy that can predict clinical outcomes, including recurrence, progression, and survival in patients with bladder cancer [29]. While E2F-5 protein is more homologous to E2F-4 (72% amino acid identity) than to E2F-1, E2F-2, and E2F-3 (35% amino acid identity), its exact role in bladder cancer is not fully understood. However, overexpression, but not point mutations, of E2F-5 has been found in breast cancer, associated with a basal phenotype and a worse clinical outcome [30].
Overexpression of E2F-6 leads to accumulation of cells in the S-phase and delays re-entry into the cell cycle in quiescent cells, thus attributing an important role for E2F-6 in the control of apoptosis via targeting of BRCA1 [31]. Its role in bladder cancer has not been fully investigated.
E2F-7 and E2F-8 are designated as atypical E2Fs because of their structural differences when compared with canonical E2Fs. E2F-7 and E2F-8, display two tandem DBDs and lack sequences that mediate Rb and DP binding (Fig. 1). The mechanisms by which atypical E2Fs regulate gene expression as well as their biological roles are still unclear, although they are able to regulate angiogenesis and lymphangiogenesis in a cell-cycle independent manner and have been implicated into E2F site-dependent transcription independent of Rb.
E2F-7 and E2F-8 share many transcriptional targets and can form homo- and heterodimers at their target promoters. E2F-7 was identified as a direct p53 target potently induced during oncogene-induced senescence [32]. By repressing mitotic genes, E2F-7 was proposed to cooperate with Rb in limiting oncogenic transformation, although its potential tumour suppressor function has not been further explored [33]. In a separate study, low mRNA levels of E2F-7 were associated with platinum resistance and reduced survival in ovarian cancer patients [34]. Surprisingly, E2F-8 behaved in the opposite direction in the same set of patients, with high levels of E2F-8 transcripts associated with poor prognosis [34]. Finally, given the crucial role of E2F-8 in angiogenesis and lymphangiogenesis [35], further studies to evaluate the contribution of E2F-8 to metastasis might bring new therapeutic opportunities for the future. Since they are the newest members of the family their role in bladder cancer has not been thoroughly investigated.
Since the discovery of microRNAs, as an important modulator for cancer development some research on miRNAs has been conducted in bladder cancer (Table 2). Thus, overexpression of miRNA-let-7a (let-7a), a microRNA shown to directly alter cell cycle progression and pro-inflammatory cytokine production, leads to increased expression of E2F-2 and Rb phosphorylation and inversely decrease of E2F-5 in immune stimulated cells [36]. Moreover, miRNA-125b inhibits the E2F-3-cyclinA2 signalling pathway and suppresses bladder tumour cells growth during G phase via the reduction of E2F-3 expression [37]. E2F-1 expression may induce oncogenic stress and promote premalignant cell apoptosis, thereby inhibiting tumour development, therefore the decrease of E2F-1 by hsa-miR-106b targeting, may be one of the possible mechanisms underlying the development of bladder cancer [38].
Control of E2Fs by microRNAs. Note that these are the miRNAs affecting only the E2F family, while others have also been implicated in bladder cancer [53].
miRNA | E2F | Effect |
---|---|---|
miRNA-let-7a | E2F-2, -5 | Up-regulates E2F-2 Downregulates E2F-5 |
miR-15 family (miR-15, 16 and 195) | E2F-3 | Downregulate |
miR-106b | E2F-1 | Downregulates |
miR-125b | E2F-3 | Deregulates |
Moreover, the novel stratification method, using molecular profiling, has identified three major subtypes of urothelial carcinoma; Urobasal (A and B), Genomically Unstable (GU) and SCC-like (SCCL). UroA and UroB tumours are molecularly similar, but UroB are biologically and clinically more progressed. GU tumours are undifferentiated, highly proliferative, while SCCL tumours show enhanced expression of basal urothelial markers and squamous differentiation. GU tumours are characterized by frequent E2F-3 gene amplifications [39]. Further analysis has revealed that E2F-1, E2F-2, E2F-3, E2F-4 and E2F-7 are highly expressed in GU and SCCL tumours [40].
Conclusions-Further perspectives
E2F family of transcription factors has been mainly implicated in cell cycle progression, classically divided in E2F activators and repressors that operate in a coordinated manner to achieve proper cell cycle progression and/or apoptosis and that disturbance of this balanced interaction can contribute to cancer development and progression. According to this model, E2Fs control the oscillating expression pattern of multiple target genes during the cell cycle: activators E2F-1-3 induce an upswing of E2F targets, essential for the G1-to-S phase transition, whereas E2F-7 and E2F-8, mediate a downswing of the same targets during late S, G2, and M phase. Unfortunately, this simplified approach, mainly stemming from in vitro studies, is challenged in practice, since no unequivocal correlation of effector and result can be easily made. To complicate things further, the plethora of potential E2F targets reflect the fact that E2Fs participate in cellular processes beyond the cell cycle; this poses a major challenge, to identify specific processes in which E2F plays a functional key role and the contexts in which a particular subset of E2F targets dictates a biologic outcome. This is crucial in developing the next generation medication to cancer, which is highly personalized and presents the minimal side-effects.
As the formerly prevailing view that E2F repressors and activators orchestrate proper cell cycle progression and disruptions in this network contribute to cancer, has now been challenged, the delineation of the complexity of E2F function and the cellular context which may dictate the different E2F roles becomes imperative. A number of key questions need to be addressed. The primary, overarching question is whether E2Fs are necessary for the proliferation of all cell types in mammals and other organisms; in case that more components are necessary, research should prioritize on identifying the essential accessories which partner with E2Fs and on how their activities are coordinated to commit cells to proliferation. A second key question is whether E2Fs enact only through their activation or repression functions and whether these activities are tailored to different developmental stages of a cell. The recent findings that E2F-1 also drives epithelial-mesenchymal transition (EMT), i.e. adopts yet another role of an inducer, similar to the classical EMT inducer TGFB1 [15] adds further complexity to the understanding of the diverse role of these proteins, rendering them as cellular mutli-tools which respond to a range of signals and stimuli. Another level of complexity is the differential expression levels of E2Fs which drive variable results. Especially in regard with bladder cancer, the number of available publications does not reflect the level of understanding of these proteins and their specific role in BC initiation or progression. In spite of the acquired knowledge on their activity, control and targets, a clear map of the full span of interactions, levels of control and differential response remains elusive.
There is now substantial evidence that of E2F-1, E2F-3 and E2F-4 and -5 have a distinct impact on bladder cancer development, progress and prognosis; so, what is the present progress in terms of translating findings into potential therapeutics? Efforts in this direction are now surfacing. CG0070 is an oncolytic adenovirus which has been engineered so that the human E2F-1 promoter drives the E1A gene, and the human GM-CSF gene is inserted. E2F-1 is regulated by the retinoblastoma tumour suppressor protein (Rb), which is commonly mutated in bladder cancer, and a loss of Rb binding results in a transcriptionally active E2F-1. A phase I trial of CG0070, conducted in patients with non-muscle-invasive bladder cancer who did not respond to BCG therapy, gave an overall response rate of 48.6% (17 of 35 recruited patients), which increased to 63.6% (14 of 22 recruited patients) in the multi-dose cohort of subjects. A later randomized phase II/III trial in patients with non-muscle-invasive bladder cancer, where 15 patients received CG0070 and 7 control patients received other standard intravesical therapies (BOND, NCT01438112), demonstrated a better durable response in a subset of high-risk patients (51), while a recent single arm phase III trial that is underway, patients with BCG-refractory non-muscle-invasive bladder cancer are given CG0070 intravesically at a dose of 1012vp weekly for 6 weeks. Patients who achieved a partial or complete response at 6 months after the first intervention are maintained with the same induction cycle every 6 months (BOND2, NCT02365818) [41].
Moreover, the connection of E2F-1 to triggering inflammatory gene expression, gives an added role to this transcription factor, since bladder cancer development is enhanced by inflammatory stimuli [42]. Fucoidan, an algae extract, increases binding of pRb to E2Fs, by blocking phosphorylation of pRb, without marked alteration of E2F-1 and E2F-4 proteins, making it an interesting candidate to anticancer therapy [43]. More recently, Palbociclib PD-0332991, which had been developed as a specific CDK4/6-inhibitor and demonstrated anti-tumour activity in preclinical models of several cancer entities including melanoma [44, 45], breast [46, 47] and ovarian cancer [48], also showed that it can reduce the proliferation of retinoblastoma positive bladder cancer cell lines and act synergistically in combination with cisplatin. Specifically, PD-0332991 or LY2835219 treatment decreased the phosphorylation, total protein and transcript level of retinoblastoma, while the treatment resulted in a decrease in E2F target gene expression (CCNA2 and CCNE2) and cell cycle progression from G0/G1 to the S-phase without affecting apoptosis. These data indicate that CDK4/6 inhibition could be a prospective therapeutic strategy for retinoblastoma positive bladder cancer that probably acts by negatively regulating retinoblastoma transcription [14].
Besides blocking of E2F, the ablation of E2F-4 -pRb interaction is currently under investigation for therapeutic use in melanomas [49], rising hopes that such an approach could be tested in bladder carcinoma as well.
The role of the remaining E2Fs remains elusive or under-investigated, despite the extensive study of all key factors, during the last decade, in urothelial development and differentiation. However, bladder cancer still remains a major health issue, with a 50 -70% recurrence and 10-20% progression to invasion. The overall 5-year survival rate for bladder cancer is 77%, and this rate drops to 34% for those with disease that has spread locally beyond the bladder and to 5% for patients with distant metastases. Therefore closer investigation of E2F factors taking into account the newer molecular stratification data as well as miRNAs development should be undertaken. Polycomb group protein Enhancer of Zest Homolog 2 (EZH2) that potentially interacts with E2F-6 is a commonly up-regulated in bladder cancer and its increased expression and activity has been associated with more aggressive forms of this cancer [50]. Besides, as described previously, the possible therapeutic use of Ezh2 inhibitors in the management of bladder cancer is a crucial aspect that merits future research.
Delineating the specific role for each E2F member and their overall contribution to bladder cancer development is a challenging task [51], especially in view of potential E2F targeted therapies. In addition to comprehensive knowledge of the E2F family functions, this necessitates the precise understanding of spatial and temporal alterations of these factors during tumour development [51], to ensure that such therapies will be successful.
Competing Interests
The authors have declared that no competing interest exists.
References
1. Charlton ME, Adamo MP, Sun L, Deorah S. Bladder cancer collaborative stage variables and their data quality, usage, and clinical implications: a review of SEER data, 2004-2010. Cancer. 2014;120(Suppl 23):3815-25
2. Antoni S, Ferlay J, Soerjomataram I, Znaor A, Jemal A, Bray F. Bladder Cancer Incidence and Mortality: A Global Overview and Recent Trends. European Urology. 2017;71(1):96-108
3. Bellmunt J. Bladder Cancer. An Issue of Hematology/Oncology Clinics of North America. 2015
4. Chen HZ, Tsai SY, Leone G. Emerging roles of E2Fs in cancer: an exit from cell cycle control. Nature Reviews Cancer. 2009;9(11):785-97
5. Gaubatz S, Lindeman GJ, Ishida S. et al. E2F4 and E2F5 play an essential role in pocket protein-mediated G1 control. Molecular Cell. 2000;6(3):729-35
6. CGARN. Comprehensive molecular characterization of urothelial bladder carcinoma. Nature. 2014;507(7492):315-22
7. Goodarzi H, Elemento O, Tavazoie S. Revealing global regulatory perturbations across human cancers. Molecular Cell. 2009;36(5):900-11
8. van den Heuvel S, Dyson NJ. Conserved functions of the pRB and E2F families. Nature reviews Molecular Cell Biology. 2008;9(9):713-24
9. Vormer TL, Hansen JB, Te Riele H. The retinoblastoma protein: multitasking to suppress tumorigenesis. Molecular & Cellular Oncology. 2015;2(1):e968062
10. Dimova DK, Dyson NJ. The E2F transcriptional network: old acquaintances with new faces. Oncogene. 2005;24(17):2810-26
11. Hurst CD, Tomlinson DC, Williams SV, Platt FM, Knowles MA. Inactivation of the Rb pathway and overexpression of both isoforms of E2F3 are obligate events in bladder tumours with 6p22 amplification. Oncogene. 2008;27(19):2716-27
12. Wang CY, Xu ZB, Wang JP, Jiao Y, Zhang B. Rb deficiency accelerates progression of carcinoma of the urinary bladder in vivo and in vitro through inhibiting autophagy and apoptosis. International Journal of Oncology. 2017;50(4):1221-32
13. Lee SR, Roh YG, Kim SK. et al. Activation of EZH2 and SUZ12 Regulated by E2F1 Predicts the Disease Progression and Aggressive Characteristics of Bladder Cancer. Clinical Cancer Research. 2015;21(23):5391-403
14. Sathe A, Koshy N, Schmid SC. et al. CDK4/6 Inhibition Controls Proliferation of Bladder Cancer and Transcription of RB1. The Journal of Urology. 2016;195(3):771-9
15. Knoll S, Emmrich S, Putzer BM. The E2F1-miRNA cancer progression network. Advances in Experimental Medicine and Biology. 2013;774:135-47
16. Lee JS, Leem SH, Lee SY. et al. Expression signature of E2F1 and its associated genes predict superficial to invasive progression of bladder tumors. Journal of Clinical Oncology. 2010;28(16):2660-7
17. Khan FM, Marquardt S, Gupta SK. et al. Unraveling a tumor type-specific regulatory core underlying E2F1-mediated epithelial-mesenchymal transition to predict receptor protein signatures. Nature Communications. 2017;8(1):198
18. Meng RD, Phillips P, El-Deiry WS. p53-independent increase in E2F-1 expression enhances the cytotoxic effects of etoposide and of adriamycin. International Journal of Oncology. 1999;14(1):5-14
19. Hayami S, Yoshimatsu M, Veerakumarasivam A. et al. Overexpression of the JmjC histone demethylase KDM5B in human carcinogenesis: involvement in the proliferation of cancer cells through the E2F/RB pathway. Molecular Cancer. 2010;9:59
20. Chang HR, Lian JD, Lo CW, Huang HP, Wang CJ. Aristolochic acid-induced cell cycle G1 arrest in human urothelium SV-HUC-1 cells. Food and Chemical Toxicology. 2007;45(3):396-402
21. Rabbani F, Koppie TM, Charytonowicz E, Drobnjak M, Bochner BH, Cordon-Cardo C. Prognostic significance of p27Kip1 expression in bladder cancer. BJU International. 2007;100(2):259-63
22. Stravopodis DJ, Karkoulis PK, Konstantakou EG. et al. Thymidylate synthase inhibition induces p53-dependent and p53-independent apoptotic responses in human urinary bladder cancer cells. Journal of Cancer Research and Clinical Oncology. 2011;137(2):359-74
23. Chen C, Wells AD. Comparative analysis of E2F family member oncogenic activity. PloS One. 2007;2(9):e912
24. Miles WO, Tschop K, Herr A, Ji JY, Dyson NJ. Pumilio facilitates miRNA regulation of the E2F3 oncogene. Genes & Development. 2012;26(4):356-68
25. Olsson AY, Feber A, Edwards S. et al. Role of E2F3 expression in modulating cellular proliferation rate in human bladder and prostate cancer cells. Oncogene. 2007;26(7):1028-37
26. Moberg K, Starz MA, Lees JA. E2F-4 switches from p130 to p107 and pRB in response to cell cycle reentry. Molecular and Cellular Biology. 1996;16(4):1436-49
27. Rayman JB, Takahashi Y, Indjeian VB. et al. E2F mediates cell cycle-dependent transcriptional repression in vivo by recruitment of an HDAC1/mSin3B corepressor complex. Genes & Development. 2002;16(8):933-47
28. Parisi T, Bronson RT, Lees JA. Inhibition of pituitary tumors in Rb mutant chimeras through E2f4 loss reveals a key suppressive role for the pRB/E2F pathway in urothelium and ganglionic carcinogenesis. Oncogene. 2009;28(4):500-8
29. Cheng C, Varn FS, Marsit CJ. E2F4 Program Is Predictive of Progression and Intravesical Immunotherapy Efficacy in Bladder Cancer. Molecular Cancer Research: MCR. 2015;13(9):1316-24
30. Umemura S, Shirane M, Takekoshi S. et al. Overexpression of E2F-5 correlates with a pathological basal phenotype and a worse clinical outcome. British Journal of Cancer. 2009;100(5):764-71
31. Yang WW, Wang ZH, Zhu Y, Yang HT. E2F6 negatively regulates ultraviolet-induced apoptosis via modulation of BRCA1. Cell Death and Differentiation. 2007;14(4):807-17
32. Carvajal LA, Hamard PJ, Tonnessen C, Manfredi JJ. E2F7, a novel target, is up-regulated by p53 and mediates DNA damage-dependent transcriptional repression. Genes & Development. 2012;26(14):1533-45
33. Aksoy O, Chicas A, Zeng T. et al. The atypical E2F family member E2F7 couples the p53 and RB pathways during cellular senescence. Genes & Development. 2012;26(14):1546-57
34. Reimer D, Sadr S, Wiedemair A. et al. Clinical relevance of E2F family members in ovarian cancer-an evaluation in a training set of 77 patients. Clinical cancer research: an official journal of the American Association for Cancer Research. 2007;13(1):144-51
35. Weijts BG, Bakker WJ, Cornelissen PW. et al. E2F7 and E2F8 promote angiogenesis through transcriptional activation of VEGFA in cooperation with HIF1. The EMBO Journal. 2012;31(19):3871-84
36. Chafin CB, Regna NL, Caudell DL, Reilly CM. MicroRNA-let-7a promotes E2F-mediated cell proliferation and NFkappaB activation in vitro. Cellular & Molecular Immunology. 2014;11(1):79-83
37. Huang L, Luo J, Cai Q. et al. MicroRNA-125b suppresses the development of bladder cancer by targeting E2F3. International Journal of Cancer. 2011;128(8):1758-69
38. Jin N, Jin X, Gu X, Na W, Zhang M, Zhao R. Screening biomarkers of bladder cancer using combined miRNA and mRNA microarray analysis. Molecular Medicine Reports. 2015;12(2):3170-6
39. Lindgren D, Sjodahl G, Lauss M. et al. Integrated genomic and gene expression profiling identifies two major genomic circuits in urothelial carcinoma. PloS One. 2012;7(6):e38863
40. Eriksson P, Aine M, Veerla S, Liedberg F, Sjodahl G, Hoglund M. Molecular subtypes of urothelial carcinoma are defined by specific gene regulatory systems. BMC Medical Genomics. 2015;8:25
41. Fukuhara H, Ino Y, Todo T. Oncolytic virus therapy: A new era of cancer treatment at dawn. Cancer Science. 2016;107(10):1373-9
42. Ghari F, Quirke AM, Munro S. et al. Citrullination-acetylation interplay guides E2F-1 activity during the inflammatory response. Science Advances. 2016;2(2):e1501257
43. Park HY, Kim GY, Moon SK, Kim WJ, Yoo YH, Choi YH. Fucoidan inhibits the proliferation of human urinary bladder cancer T24 cells by blocking cell cycle progression and inducing apoptosis. Molecules (Basel, Switzerland). 2014;19(5):5981-98
44. Young RJ, Waldeck K, Martin C. et al. Loss of CDKN2A expression is a frequent event in primary invasive melanoma and correlates with sensitivity to the CDK4/6 inhibitor PD0332991 in melanoma cell lines. Pigment Cell & Melanoma Research. 2014;27(4):590-600
45. Yadav V, Burke TF, Huber L. et al. The CDK4/6 inhibitor LY2835219 overcomes vemurafenib resistance resulting from MAPK reactivation and cyclin D1 upregulation. Molecular Cancer Therapeutics. 2014;13(10):2253-63
46. Finn RS, Dering J, Conklin D. et al. PD 0332991, a selective cyclin D kinase 4/6 inhibitor, preferentially inhibits proliferation of luminal estrogen receptor-positive human breast cancer cell lines in vitro. Breast Cancer Research: BCR. 2009;11(5):R77
47. Dean JL, Thangavel C, McClendon AK, Reed CA, Knudsen ES. Therapeutic CDK4/6 inhibition in breast cancer: key mechanisms of response and failure. Oncogene. 2010;29(28):4018-32
48. Konecny GE, Winterhoff B, Kolarova T. et al. Expression of p16 and retinoblastoma determines response to CDK4/6 inhibition in ovarian cancer. Clinical Cancer Research. 2011;17(6):1591-602
49. Ma Y, Kurtyka CA, Boyapalle S. et al. A small-molecule E2F inhibitor blocks growth in a melanoma culture model. Cancer Research. 2008;68(15):6292-9
50. Santos M, Martinez-Fernandez M, Duenas M. et al. In vivo disruption of an Rb-E2F-Ezh2 signaling loop causes bladder cancer. Cancer Research. 2014;74(22):6565-77
51. Evangelou K, Havaki S, Kotsinas A. E2F transcription factors and digestive system malignancies: how much do we know? World Journal of Gastroenterology. 2014;20(29):10212-6
52. Attwooll C, Lazzerini Denchi E, Helin K. The E2F family: specific functions and overlapping interests. The EMBO Journal. 2004;23(24):4709-16
53. Bueno MJ, Malumbres M. MicroRNAs and the cell cycle. Biochimica et Biophysica Acta. 2011;1812(5):592-601
54. Morgunova E, Yin Y, Jolma A. et al. Structural insights into the DNA-binding specificity of E2F family transcription factors. Nature Communications. 2015;6:10050
Author contact
Corresponding author: Kalliopi Kostelidou, Bone Marrow Transplant Unit, Haematology-Lymphoma Clinic, Evangelismos General Hospital, Ypsilantou 45-47, GR10676 Athens, Greece. Tel: +30 213 204 3253, +306982243439 Email: kkostelidougr, diplokappacom
Received 2017-7-26
Accepted 2018-7-29
Published 2018-10-31